Experimentally realizing novel quantum phases of matter and manipulating their properties is a central goal of modern condensed-matter physics, where utilizing ultrafast light-matter interaction is one of the emerging techniques, which holds promise for a new generation of electronic/photonic devices. However, inducing permanent metastable electronic states in a fully reversible manner remains a long-standing challenge. Recently, Professor Nanlin Wang’s research group and their collaborators achieved all-optical manipulation of electronic polar states in quasi-two-dimensional (2D) charge density wave (CDW) system EuTe4 using ultrafast lasers. Their study demonstrated reversible non-volatile changes of second harmonic generation (SHG) signal and resistance at room temperature, where layer-specific phase inversion of the Te-layers and their various stacking orders may be the key factor. This work was published in the journal Nature Communications on October 17, 2024.
EuTe4, first discovered by associate research scientist Dong Wu in prof. Nanlin Wang's group in 2019, is a rare CDW semiconductor due to the concomitant formation of in-plane polarization. Its crystal structure consists of planar Te-sheets separated by insulating Eu-Te slabs (Fig. 1a), and it forms a 1a×3b×2c superlattice (point group C2v) due to CDW distortion at room temperature. In a single Te layer, the distortion of Te atoms along the a-axis leads to the formation of regular Te trimers, which collectively generates a net polarization e along the a-axis, perpendicular to the CDW modulation wave vector q (along the b-axis, Fig. 1b). The parallel in-phase stacking of polarization in adjacent Te-sheets results in spatial inversion symmetry breaking along the a-axis and hence a polar order in EuTe4 (Fig. 1c). Consistent with previous transport, ARPES and XRD results [1-3], the temperature-dependent SHG intensity exhibits an unconventional thermal hysteresis (Fig. 1e) and it is interpreted as arising from unusual switching of the relative CDW phase in different Te layers.
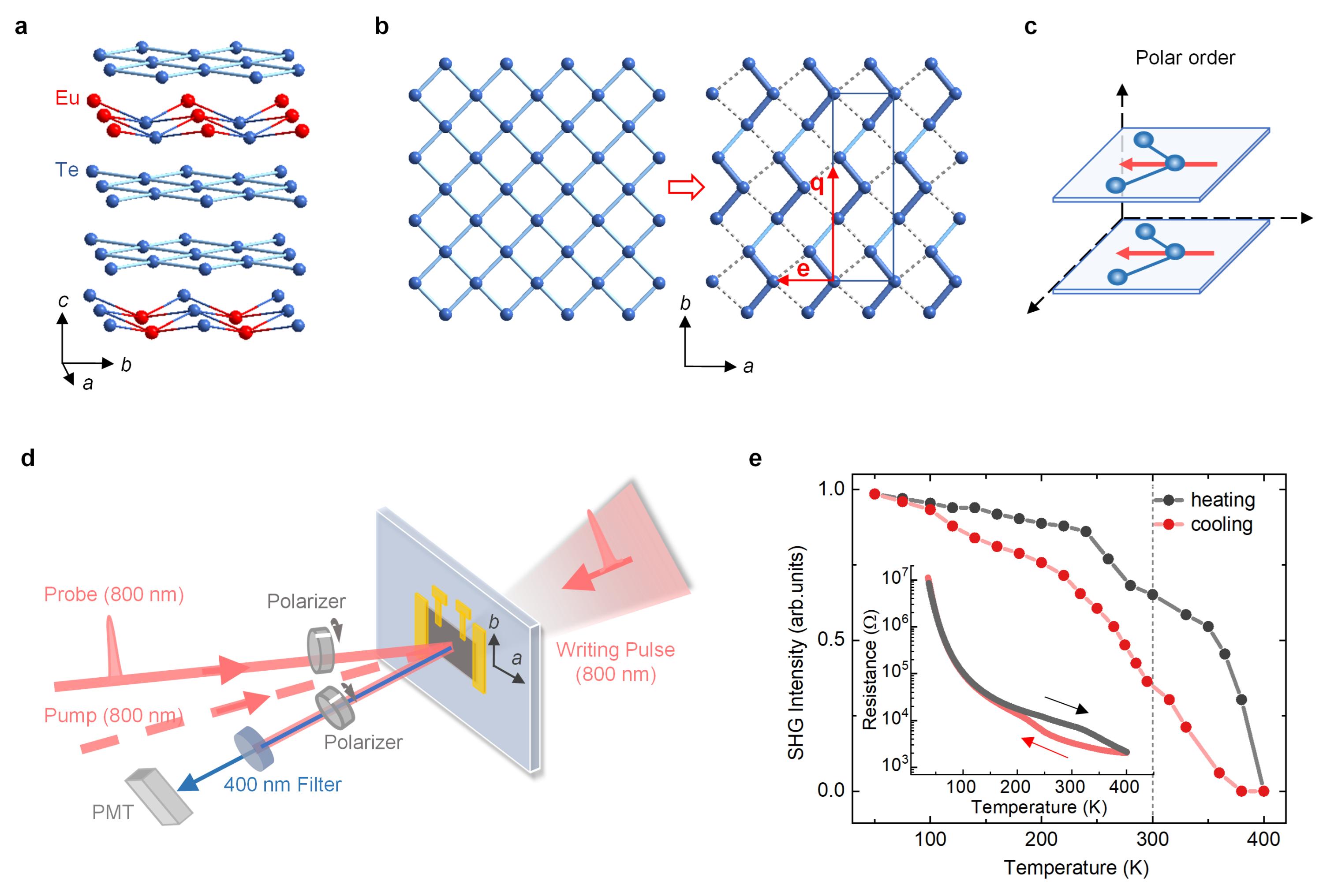
Fig. 1| a. The averaged crystal structure of EuTe4. b. The formation of Te-trimers and polarization. The CDW vector q is oriented along the b-axis, which is perpendicular to the polarization vector e. c. The schematic of stacking sequence of the polarized Te-sheets for the formation of polar order, where Te trimers in adjacent Te-sheets align in the same direction. d. A Sketch of the experimental setup. e. Temperature dependence of SHG intensity of the pristine sample.
Combining 800 nm ultrafast laser excitation, SHG and electrical measurements at room temperature, the research team finds that a single writing pulse can enhance or suppress the SHG intensity and resistance of nm-thick EuTe4 samples. There are two distinct excitation regimes separated by a threshold fluence of writing pulse (FcR ~ 6.8 mJ/cm2). In regime-Weak (Fig. 2), non-volatile changes can be induced within the system’s hysteresis. Starting from the initial state at 300 K on the cooling branch, weaker pulses enhance both the SHG and resistance (1.5 mJ/cm², Fig. 2a, b), while stronger pulses (2.5 mJ/cm², Fig. 2c, d) are required to suppress the SHG and resistance if starting from the initial heating branch state. In regime-Strong (Fig. 3), a single writing pulse creates a new non-volatile state with no SHG signal but with resistance increase by several orders of magnitude. Subsequently, a train of thousands of moderate pulses (in a range of 4-5 mJ/cm2), induces a new polar state characterized by low resistance and a unique SHG pattern. Analysis of this SHG pattern reveals a strongly enhanced second-order susceptibility tensor component caaa.
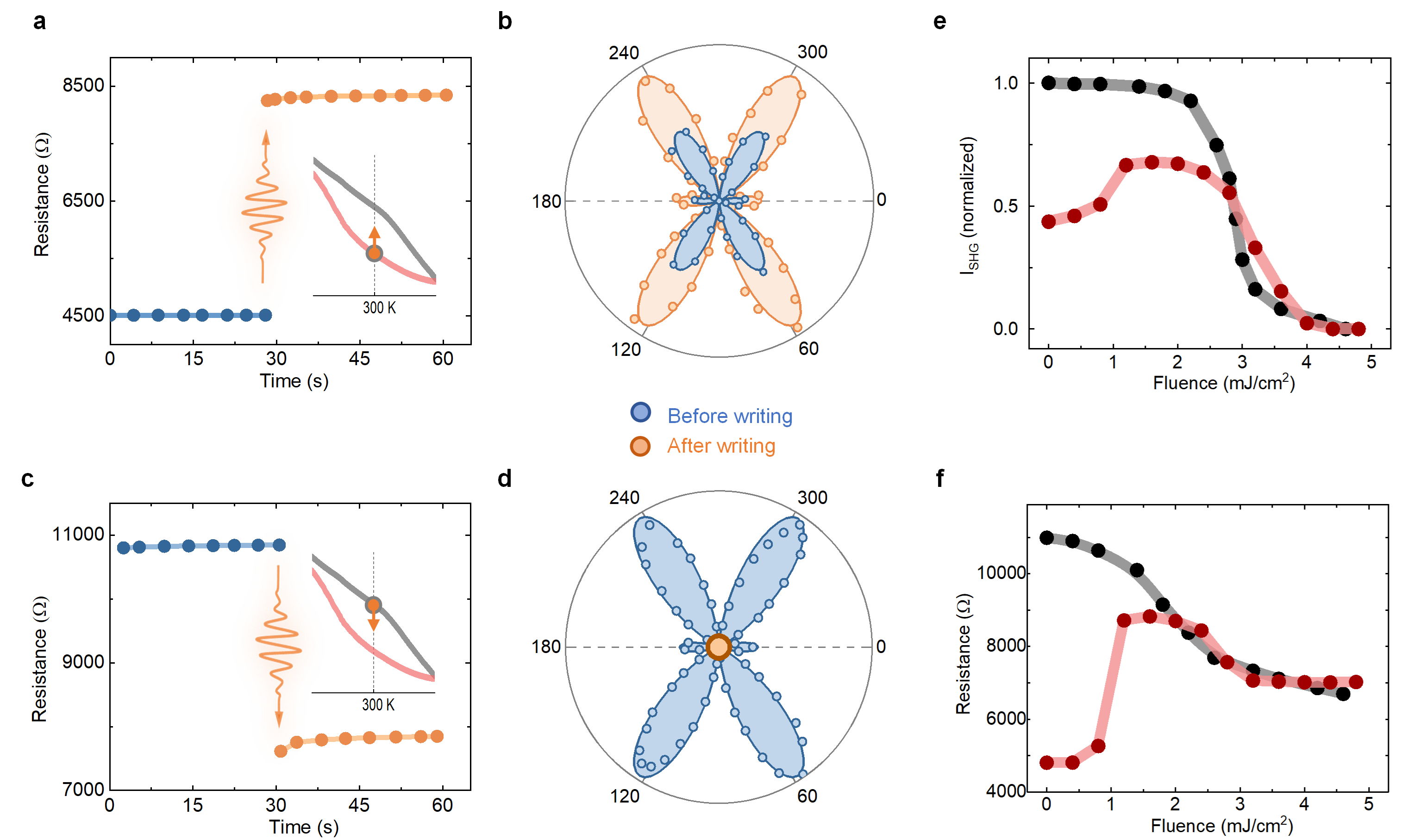
Fig. 2 |Non-volatile switching of SHG intensity and resistance in regime-Weak. a, b. Starting from the state at 300 K on cooling branch (see the inset in (a)), a single weak pulse, e.g., 1.5 mJ/cm2, enhances both resistance and SHG intensity. c, d. Conversely, at 300 K on heating branch, a more intense pulse, e.g., 4.5 mJ/cm2, suppresses both resistance and SHG intensity. e, f. Shot-to-shot SHG intensity and resistance as a function of writing fluence. The data points are measured by increasing fluence in sequence starting from the cooling (red curves) or heating branch (black curves) respectively.
The photo-induced permanent change in SHG intensity reflects the change of the material’s polarization. In view of layered structure of EuTe4, there are six inequivalent Te layers stacking alternately along the c-axis in a CDW supercell, and each Te-layer exhibits electric polarization along the a-axis, either positive or negative in principle, depending on the direction of the CDW distortion. The overall polarity in EuTe4 is thus determined by the relative stacking order of these Te-layers, potentially resulting in various spatial symmetries and multiple polar phases. For instance, in addition to the polar phase, an antipolar phase with inversion symmetry is also reasonable based on our crystallographic modeling, which uses the same XRD data obtained from pristine samples. In this scenario, the system’s overall behavior mirrors that of conceptual anharmonic two-level system through phase inversion: higher fluences tend to excite more states with weaker averaged electric polarization, while lower fluences lead the excited states back into polar phases with stronger electric polarization by overcoming shallow barriers. Furthermore, the new phase observed in regime-Strong is expected to involve additional lattice distortion. Further studies are needed to confirm this proposed mechanism of layer-specific polarization inversion and the lattice distortion through more direct measurement techniques such as XRD or transmission electron microscopy.
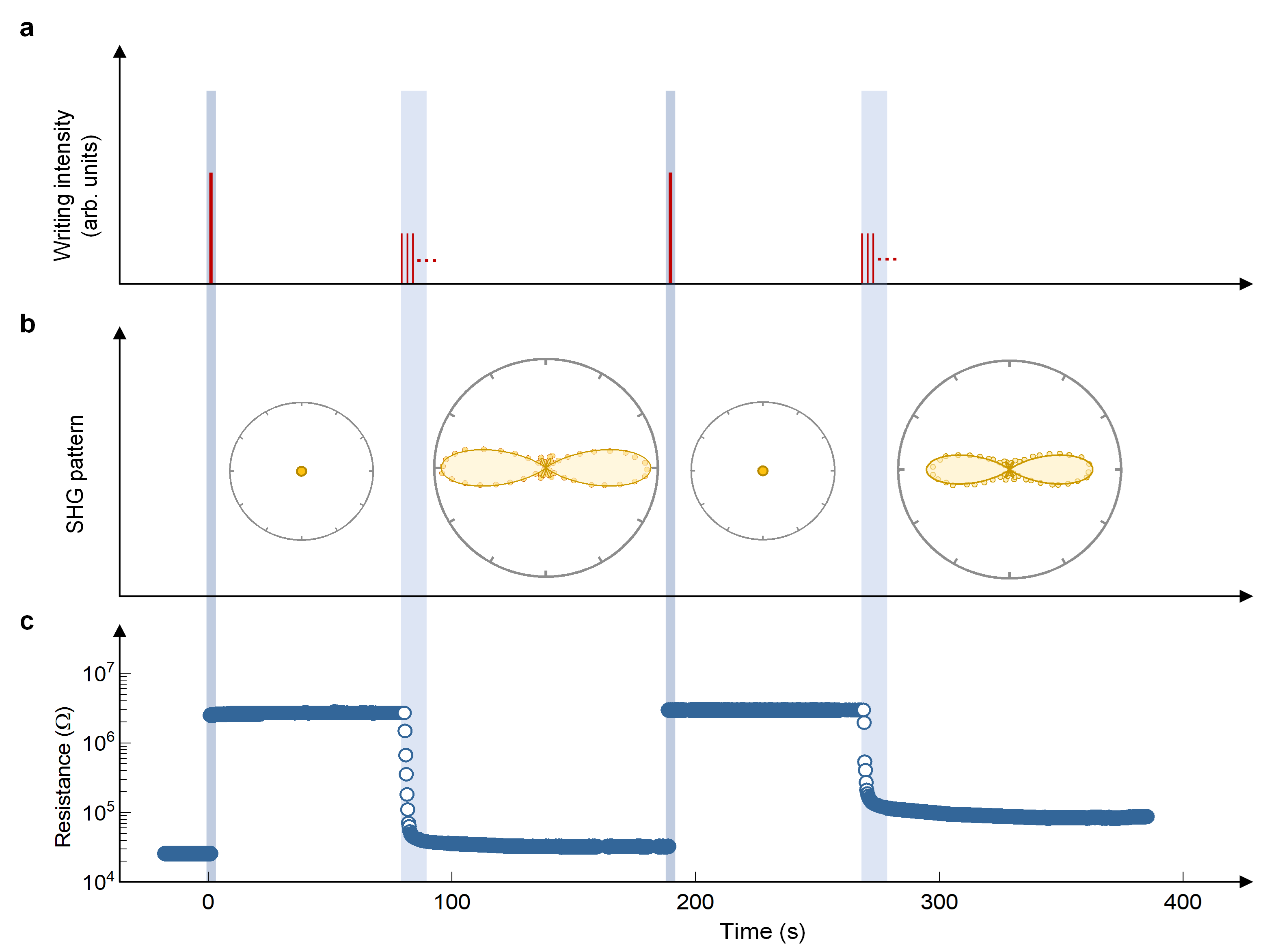
Fig. 3 Non-volatile switching of SHG intensity and resistance induced in regime-S. a. Depiction of the writing pulse number and intensity used in sequence: a single shot with strong excitation intensity of about 7.5 mJ/cm2, and the subsequent ten thousand shots (10 s, 1 kHz) with moderate intensity of ~4.5 mJ/cm2. b. The measured SHG pattern and intensity switching after exposure to the writing pulses corresponding to (a). A new SHG pattern is induced under the moderate excitations. c. The corresponding sharp resistance switching.
In this work, the Boya postdoc Qiaomei Liu at ICQM and associate research scientist Dong Wu at BAQIS in Prof. Nanlin Wang’s group are the co-first authors. Dong Wu and Nanlin Wang are the corresponding authors. This work was supported by National Natural Science Foundation of China, the National Key Research and Development Program of China and the Office of Science, U.S. Department of Energy.
Paper Link:https://www.nature.com/articles/s41467-024-53323-0