A quantum computer works based on qubits. By manipulating quantum states to realize specific logical operation, quantum computing can solve some important computing problems that cannot be effectively completed by classical computers, which may have a decisive role in future information technology and, thus, has attracted widespread attention worldwide. The fascinating prospect for quantum computing in application has inspired international high-tech companies, e.g. Google, IBM, Microsoft, Intel, Alibaba, Tencent, Baidu, and many start-ups, to invest heavily in related research. Meanwhile, the European Union has launched a �1 billion quantum flagship, and U.S., Britain, Germany, Japan, etc., have also made important strategic layouts in the field of quantum information science.—The world has entered the times of so-called quantum supremacy. The main problem for classical quantum computing is the decoherence of qubits, and the derived low fidelity as increasing qubits number, which requires more qubits for error correction. Therefore, exploring fault-tolerant quantum computing, or equivalently, topological quantum computing that is insensitive to environmental noises, has become an important route towards large-scale quantum computing.
Majorana fermion is distinctive with antiparticle being itself, and has never been captured in particle physics. The ‘counterpart’ in condensed matter, Majorana quasiparticle, behaves as Majorana zero-energy bound state (ZEBS) or Majorana zero mode (MZM), in zero-dimension. MZM obeys non-Abelian statistics, and is immune to local perturbation with high fault tolerance, which has been intensively investigated as a potential building block for topological qubit. Based on resonant Andreev reflections, the typical signal of MZM in tunneling experiments is zero-bias conductance peak (ZBCP). Theoretically, MZM is predicted to exist in the ends/topological defects (e.g. magnetic vortex) of strong Rashaba spin-orbit coupled nanowires, topological insulators, and spin-textured Fe atomic chains in proximity to s-wave superconductors. Based on these proposals, the ZEBSs consistent with theoretical expectations have been experimentally detected. Although the reported evidence for MZM is not fully accepted by all experts, the relevant experimental progress always attracts widespread attention from the international academic and industrial communities. However, the nano fabrication technology, the ultralow temperature, and the necessity of external magnetic fields required for MZM implementations make the possible application of MZM highly challenging. Recently, iron-based superconductor, bulk Fe(Te,Se), has been demonstrated with superconducting topological surface states, probably providing alternative directions for addressing the above issues. However, its relatively low superconducting transition temperature Tc (~15 K) and the difficult-to-control character of magnetic vortices therein require optimized MZM physical settings in this direction to explore the possibility of MZM in high-temperature superconductors.
Recently, Jian Wang group at Peking University, in collaboration with Prof. Ziqiang Wang at Boston College and Prof. Jiangping Hu at Institute of Physics, Chinese Academy of Sciences, detected novel ZEBSs resembling the characteristics of MZMs in interstitial Fe adatoms deposited on the high-temperature superconducting thin films at two-dimensional limit.
By ultrahigh vacuum molecular beam epitaxy, Jian Wang group successfully prepared the macro-scale, single-layer-thick (thickness < 1 nm) high-temperature superconductors fese and fete0.5se0.5 single crystal thin films on srtio3 substrates, showing transition temperature tc of about 60 k. previously, by using in situ scanning tunneling microscopy/spectroscopy, jian wang group has studied their superconducting pairing mechanisms [prl 123, 036801 (2019); nano letters 19, 3464 (2019); nano letters 20, 2056 (2020)]. on this basis, jian wang group deposited fe adatoms on the surface of single-layer fese and fete0.5se0.5 films by molecular beam epitaxy (substrate temperature: ~143-155 k; adatom coverage: ~0.002-0.003 layer). in situ scanning tunneling microscopy observations show that the deposited fe adatoms are located at the interstitial hollow site of four adjacent te/se atoms in the upper sub-layers of the films. due to the ultralow adatom deposition density, the fe adatoms exist as isolated individual adsorbed atoms without neighboring fe adatom clusters nearby. systematic experiment of in situ ultrahigh vacuum (~10-10 mbar) scanning tunneling spectroscopy reveals that, for specific coupling strength between adatoms [amounting to ~13% (~15%)] and single-layer fese (fete0.5se0.5), sharp zbcps can be observed on the fe adatoms (figure 1). the zbcp is closely distributed near the adatom site with decay length of ~3a, and unsplit when moving away from the adatom center. temperature-dependent experiments show that the zbcp disappears far below the superconducting transition temperature, which can preliminarily exclude the interpretations based on kondo effect and conventional impurity scattering states (figure 2a and figure 2b). further control experiments and analyses indicate that, the zbcp: a) shows the full-width at half maximum strictly limited by temperature and instrumental broadenings, b) does not split in fe adatom dimer, and c) obeys the majorana scaling equation. all these characteristics resemble the phenomenological signatures of mzm (figures 2c-2g). basically, the fe adatoms deposited on single-layer fese and fete0.5se0.5 films share nearly the same spectroscopic results. the statistical results suggest that, compared with the case in single-layer fese, the fe adatoms on single-layer fete0.5se0.5 show higher zebs probability and stronger zebs signal.
Prof. Wang Ziqiang and collaborators have theoretically proposed that, in the absence of an external magnetic field, the interstitial magnetic impurities in strong spin-orbit coupling s-wave superconductors can generate quantum anomalous vortices. In theory, if single-layer FeSe and FeTe0.5Se0.5 have strong Rashba spin-orbit coupling due to inversion symmetry breaking, and the magnetic moments of Fe atoms locally break the time-reversal symmetry, the quantum anomalous vortices can support MZMs. Some theories also predict the existence of topologically nontrivial phases in single-layer FeSe and FeTe0.5Se0.5. In a two-dimensional topological superconductor, the MZM can also arise in a quantum anomalous vortex nucleated at an Fe adatom. Therefore, the ZBCP observed in our experiment can be attributed to the the emergence of quantum anomalous vortices at the Fe adatoms. A deeper and more detailed understanding would need further experimental and theoretical explorations. This work extends the superconducting materials for MZM explorations from three-dimension to two-dimension, and from low-temperature to Tc > 40 K high-temperature superconductors. Additionally, no external magnetic field is needed for inducing the ZEBS, and the ZEBS can be manipulated in principle, and the ‘surviving’ temperature is also significantly increased, which provide possible solutions for the realization of applicable topological qubits in the future.
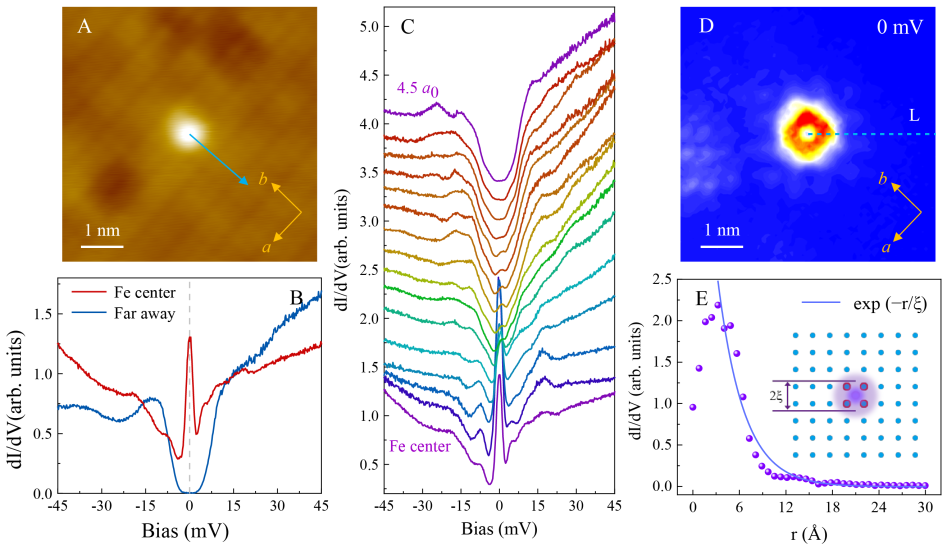
|
Figure 1. A,B, Isolated Fe adatom and the tunneling spectra on single-layer FeSe. C, Tunneling spectra collected along the arrow in A. D, Zero-bias conductance imaging for the adatom in A. E, Exponential fit to the linecut L in D. |
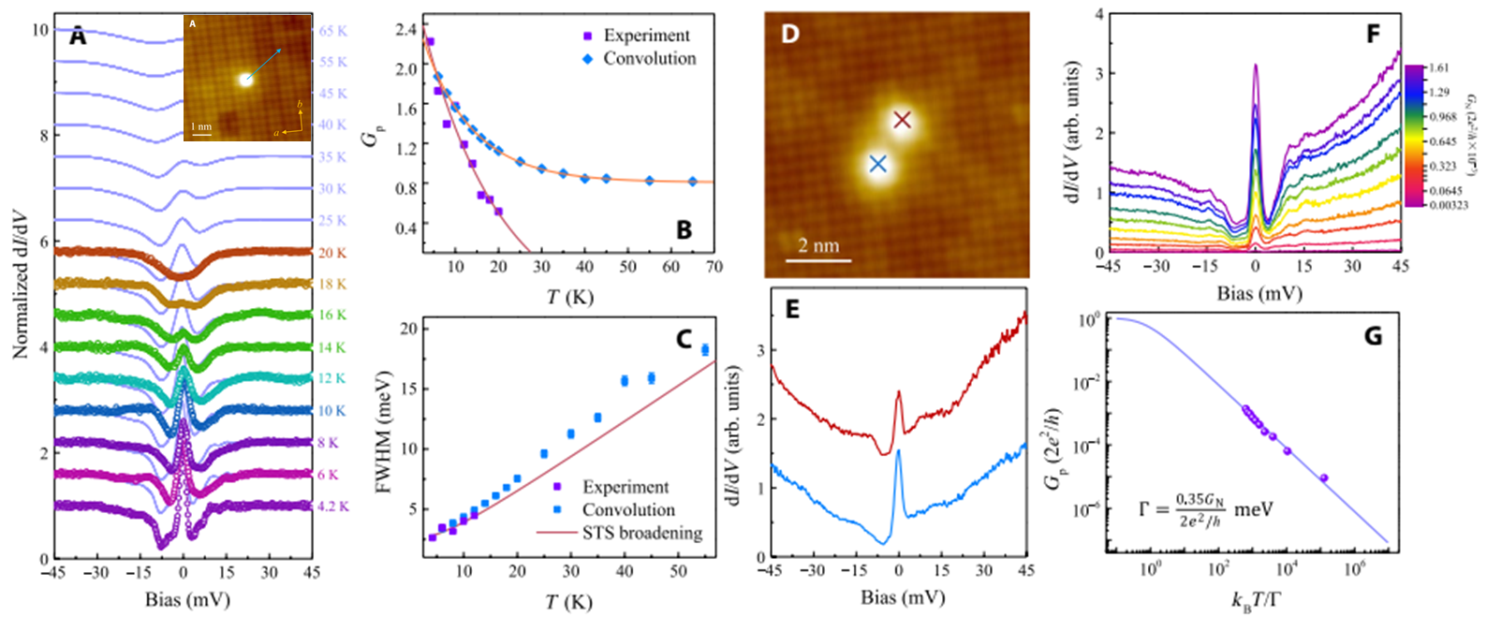
|
Figure 2. A, Temperature-dependent tunneling spectra of the ZEBS induced by Fe adatoms on single-layer FeTe0.5Se0.5 (open circles). The solid curves are the convoluted 4.2-K spectra by Fermi-Dirac distribution function at higher temperatures (both vertically offset for clarity). The inset is the atomic resolution image of Fe adatom on single-layer FeTe0.5Se0.5. B, The temperature evolution of the zero-bias conductance Gp extracted from A. C, Width analysis of ZBCPs. D,E, Fe adatom dimer and corresponding tunneling spectra. F, The ZEBS spectra at different tunneling-barrier conductances GN. G, Majorana scaling equation analysis. |
The work was published on Science Advances [Sci. Adv. 6: eaax7547 (2020)] on March 25, 2020. Prof. Jian Wang at Peking University is the corresponding author, and Chaofei Liu and Cheng Chen at Peking University are the co-first authors. The theoretical collaborators are Prof. Ziqiang Wang at Boston College and Prof. Jiangping Hu at Institute of Physics, Chinese Academy of Sciences. This work is supported by the National Natural Science Foundation of China, the National Key Research and Development Program of China, the Collaborative Innovation Center of Quantum Matter, the Strategic Priority Research Program of Chinese Academy of Sciences, Beijing Natural Science Foundation, and the US Department of Energy, Basic Energy Sciences.