Scanning Tunneling Microscope (STM) is able to visualize individual atoms at surfaces because of the highly localized tunneling current. However, due to the limitation of the bandwidth of the current amplifier, the temporal resolution of STM is usually at the order of microsecond (μs, 10-6 s), far slower than most of the microscopic dynamic processes, which are on the order of picosecond (ps, 10-12 s) and femtosecond (fs, 10-15 s). A feasible way to achieve “Femtosecond-Angstrom” resolution is combining the optical pump-probe technique with the STM. While the scheme has been proposed since 1990s, the progress towards this direction was greatly hindered by a series of technical difficulties, e.g., the disturbance of the tunneling current resulting from the thermal effect of the laser illumination, the low signal-to-noise ratio (SNR) of the laser-induced current, the broadening of the pulse width in the STM, the coupling mechanism between the laser and tunneling electrons, etc. Now, the team led by Ying Jiang and Enge Wang of International Center for Quantum Materials (ICQM) of Peking University, in collaboration with Sheng Meng and Yuxiang Weng of Institute of Physics (IOP), Chinese Academy of Sciences (CAS), successfully developed an ultrafast laser-combined STM that simultaneously achieves femtosecond temporal resolution and atomic-scale spatial resolution, and captured the non-equilibrium dynamics of a single polaron on a metal-oxide surface. This work was published inPhys. Rev. Lett. 124, 206801 (2020)on May 19, 2020 and was selected as an Editors’ Suggestion.
Jiang Group has started constructing the ultrafast laser-combined STM since 2012. All the key components were home-made, including the scanner, the vacuum system, the STM controller and the laser-coupling system. After persistent efforts for more than 5 years, the team finally completed a new-generation ultrafast laser-combined STM (Figure 1), which allows to probe ultrafast dynamics at the atomic scale. By developing the specially designed scanner and the laser modulation technique, the researchers effectively suppressed the laser thermal effect and greatly enhanced the light-induced signals. The system can work under ultrahigh vacuum (UHV) and liquid-helium temperature, with the best temporal resolution of 180 fs and the pump-probe delay range reaching several μs.
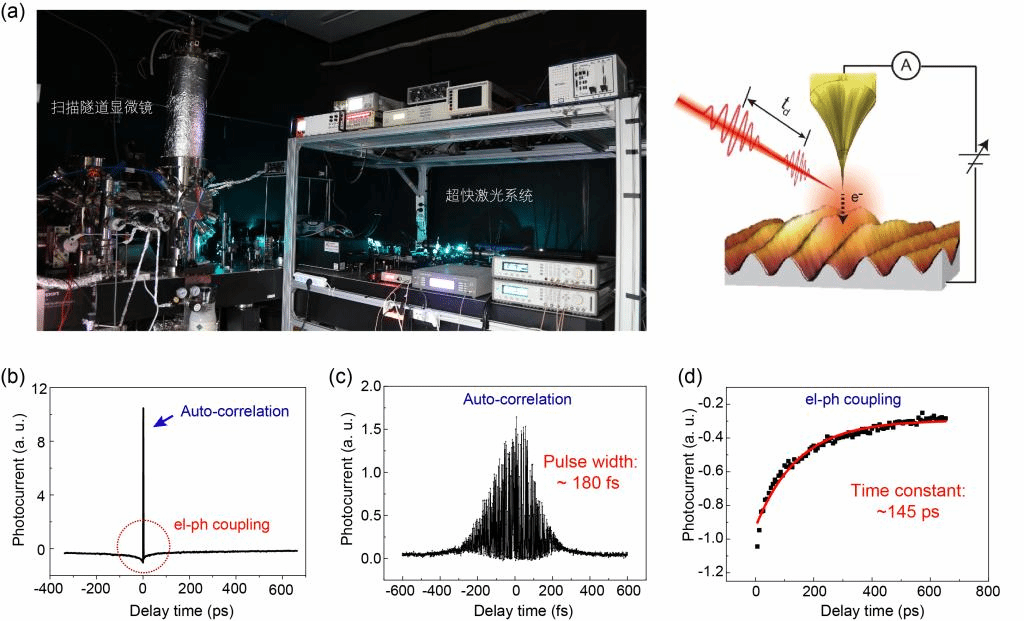
|
Figure 1. (a) Ultrafast laser-combined scanning tunneling microscope. (b) Laser-induced tip photocurrent versus delay time between pump and probe pulses. (c) Auto-correlation of the photocurrent, indicating a temporal resolution better than 180 fs. (d) Photocurrent versus time delay outside the interference region, indicating a time constant of 145 ps, which corresponds to the suppression of the photocurrent by the electron-phonon coupling.
|
Using this new instrument, the researchers succeeded to unravel the non-equilibrium dynamics of a single polaron with atomic precision (Figure 2). Polarons play a critical role in a wide spectrum of physicochemical properties of many metal oxides, such as photocatalysis, high-Tc superconductivity, thermoelectricity, and colossal magnetoresistance, to name just a few. Taking a prototypical photocatalyst—titanium dioxide (TiO2), for example—the formation of oxygen vacancies in metal oxides introduces excess electrons into the surrounding lattice, leading to polarons bound to the oxygen vacancies. Such polarons are responsible for the localized states within the band gap. Probing the non-equilibrium dynamics of these photoexcited polaron states at the atomic scale is the key to achieving a microscopic understanding of various photoconversion processes.
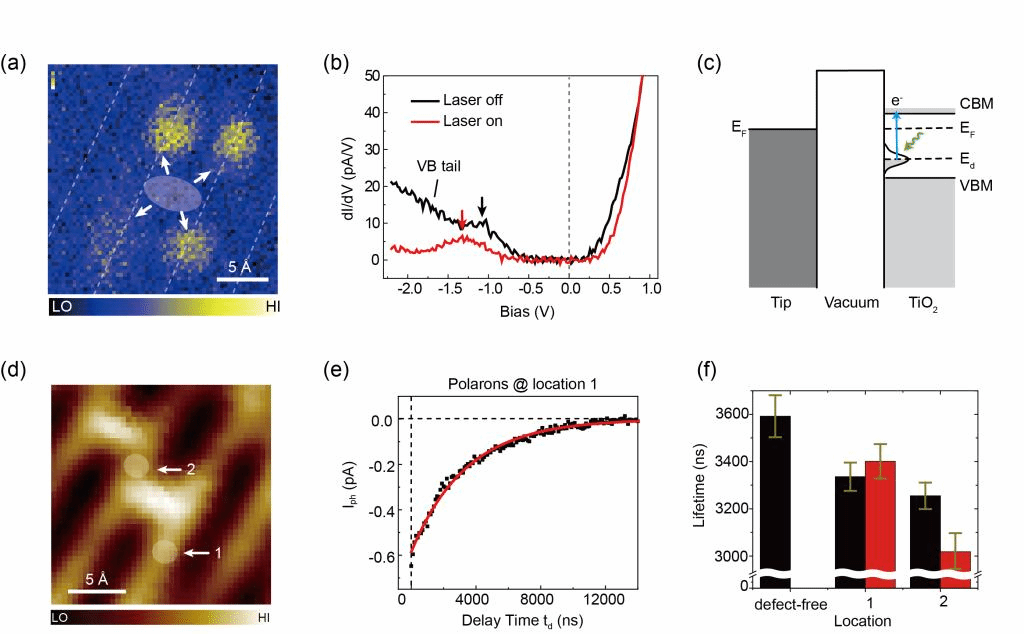
|
Figure 2. (a) Distribution of polaron states around an oxygen vacancy. (b) Scanning tunneling spectroscopy at the polaron site with laser on/off, indicating that electrons are photoexcited from the polaron states to the conduction band (c). (d) The topography of an oxygen vacancy dimer, where two polaron sites are denoted. (e) Relaxation dynamics of the polaron at location 1 in (d). (f) Location dependence of the lifetimes for photoexcited electrons (black) and polarons (red).
|
In their study, the researchers revealed that the visible-light excitation of the polarons leads to depletion of the polaron states and delocalized free electrons in the conduction band, while in the relaxation process the electrons are trapped by oxygen vacancies to form bounded polarons again. By probing the relaxation dynamics of the single polaron, the researchers found that the polaron trapping becomes considerably faster when the polaron is bound to two surface oxygen vacancies than that to one. However, the lifetime of the electrons is less sensitive to the atomic-scale aggregation of the defects but exhibits more prominent dependence on the nanoscale density fluctuation of the defects.
This work provides the first spatiotemporal information for the relaxation dynamics of photoexcited single polarons, which reveals the critical role of atomic environments. It may also modify the conventional picture of photocatalytically active sites at the metal-oxide surface and provide new guides for defect engineering of photocatalytic materials. Furthermore, the techniques and methods developed in this work provide further possibility for studying the microscopic carrier dynamics in materials, such as photoelectric conversion, exciton dynamics, charge transport and electron-phonon coupling.
Chaoyu Guo, Xiangzhi Meng, Qin Wang of ICQM and Huixia Fu of IOP are the co-first authors of this work. Ying Jiang, Enge Wang and Sheng Meng are the co-corresponding authors. This work received supports from National Natural Science Foundation of China, Ministry of Science and Technology of China, Chinese Academy of Sciences, and Beijing Municipal Science and Technology Commission.