Optical sensing of nanoscale objects with ultrahigh sensitivity is highly
desirable for applications in various fields, such as in early-stage diagnosis
of human diseases and in environmental monitoring, as well as in homeland
security. The ultimate sensitivity of a nanoparticle sensor is expected to be at
the level of asingle nanoparticle response.Very recently, a group led by Prof.
Yun-Feng Xiao and Prof. Qihuang Gongfrom Peking University, has developed a
novel microcavity Raman-lasersensor, and realized single nanoparticle detection
in both air and in an aqueous environment. The smallest nanoparticle they
detected in water was 20 nanometers in radius. Their new experimental results
were publishedin Proceedings of National Academy of SciencesonlineEarly Edition
(DOI: 10.1073/pnas.1408453111).
The microcavities they use are called whispering gallery mode (WGM) optical
microcavities, which are analogous to the acoustic whispering gallery in St.
Paul Cathedral in London and the echo wall in the Temple of Heaven in Beijing.
Due to the lowpropagation loss and small size of such microcavities, photons can
circulate inside for up to hundreds of thousands of times, thus dramatically
increasing light-matter interactions. Therefore, WGM microcavities areideal
platforms for label-free, ultrasensitive detection of single nano-objects.
In this work, researchersdetected nanoparticles using stimulated Raman
scattering (also called Raman lasing) in the microcavities.Raman scattering is
an inelastic light scattering process, whereby the energy of anincident photonis
modified by an inelastic interaction with the cavity material. The cavity
material changes its energy state and the photon loses an equivalent amount of
energy. The scattered light has a lower frequency and is called the Stokes
emission.The energy difference between the incoming photon and the scattered
Stokes photon matches a molecularvibration in the cavity material, as shown in
Figure 2(a).A typical Raman spectrum of the silica microcavity is plotted in
Figure 2(b), with the pump in the 680 nm wavelength band.
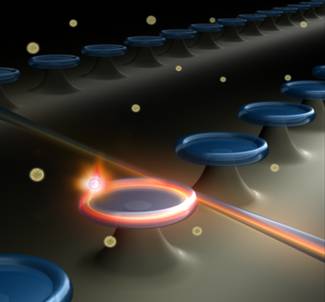
|
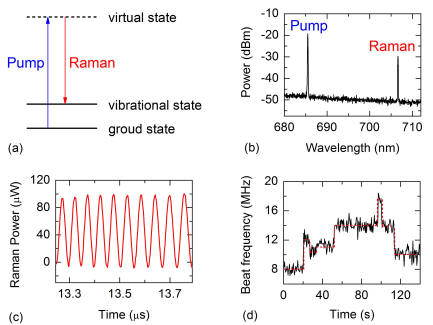
|
Figure 1. An Array of microcavity Raman lasers for single
nanoparticle detection. The pump laser light (in orange) is coupled into
the microcavity viaa fiber taper to generate the Raman laser (in red).
When the Raman laser encounters a nanoparticle, it splits into two
lasing lines due to backscattering, thus producing a beat note in the
temporal Raman power. By measuring the beat frequency changes, real-time
single nanoparticle detection can be performed.
| Figure 2. (a), Schematic of the Stokes Raman scattering process.
(b), A typical Raman spectrum of the silica microcavity with the pump in
the 680 nm wavelength band. (c), The Raman power in the time domain,
which shows a beat oscillation,with the beat frequency being the sensing
signal for single nanoparticle detection. (d),The beat frequency change
induced by single nanoparticle binding events.
|
Stimulated Ramanscattering occurs in both counter-propagating traveling
cavity modes(i.e., clockwise and counterclockwise modes) which are initially
degenerate. When the Raman laser encounters a nanoscale object,it will split
into two Raman lines, because the nano-object induces a coupling between the two
counter-propagating modes through backscattering. When both split modes lase
simultaneously, a beat note is produced (as shown in Figure 2(c)), with the beat
frequency corresponding to the Raman mode splitting. Thus,by monitoring the beat
frequency of the split-mode Raman lasers,ultrasensitive nanoparticle detection
can be realized, as shown in Figure2(d) of the above figure.
This mode splitting sensing mechanism is robust to many noise sources, such
as thermal noise and laser frequency noise, since the two split modes share
exactly the same noise. Moreover, Raman laser has much narrower linewidth
compared to a passive microcavity, and thus to significantlylower the detection
limit.In order to demonstrate the feasibility of this sensing mechanism, the
researchers first did a control experiment in air. They transferred single
nanoparticles to and from the microcavity using a nanofiber, using a technology
previously developed by the same group, and measured the beat frequency changes
after each transfer process. As expected, every time a nanoparticle is
transferredto the cavity surface, the beat frequency of the Raman laser
experienced an abrupt change. Using this method, real-time single nanoparticle
detection in an aqueous environment was then performed, with the smallest
particle of 20 nanometers in radius detected.
Another advantage of this sensing mechanism is that Raman gain is an inherent
property of almost any material, so we do not need to dope the cavity with an
additional gain medium. Furthermore, since Raman scattering occurs under
practically any pump wavelength, this sensing method also loosens the
requirement of specific wavelength bands for the pump laser. Due to these
advantages, this Raman-laser sensor represents a significant step towards
practical microlaser sensors.